Table of Contents Hide
- 1 An LED Light Is Only As Good As Its Driver
- 2 What Is an LED Driver?
- 3 Constant Current or Constant Voltage?
- 4 Switched-mode Power Supply (SMPS)
- 5 Linear Power Supply
- 6 Switched Vs. Linear
- 7 DOB
- 8 Power Utilization
- 9 Dimming Control
- 10 Flicker Mitigation
- 11 Circuit Protection
- 12 EMI and EMC
- 13 Safety Considerations
- 14 Thermal Considerations
- 15 Ingress Protection
- 16 Location Impact
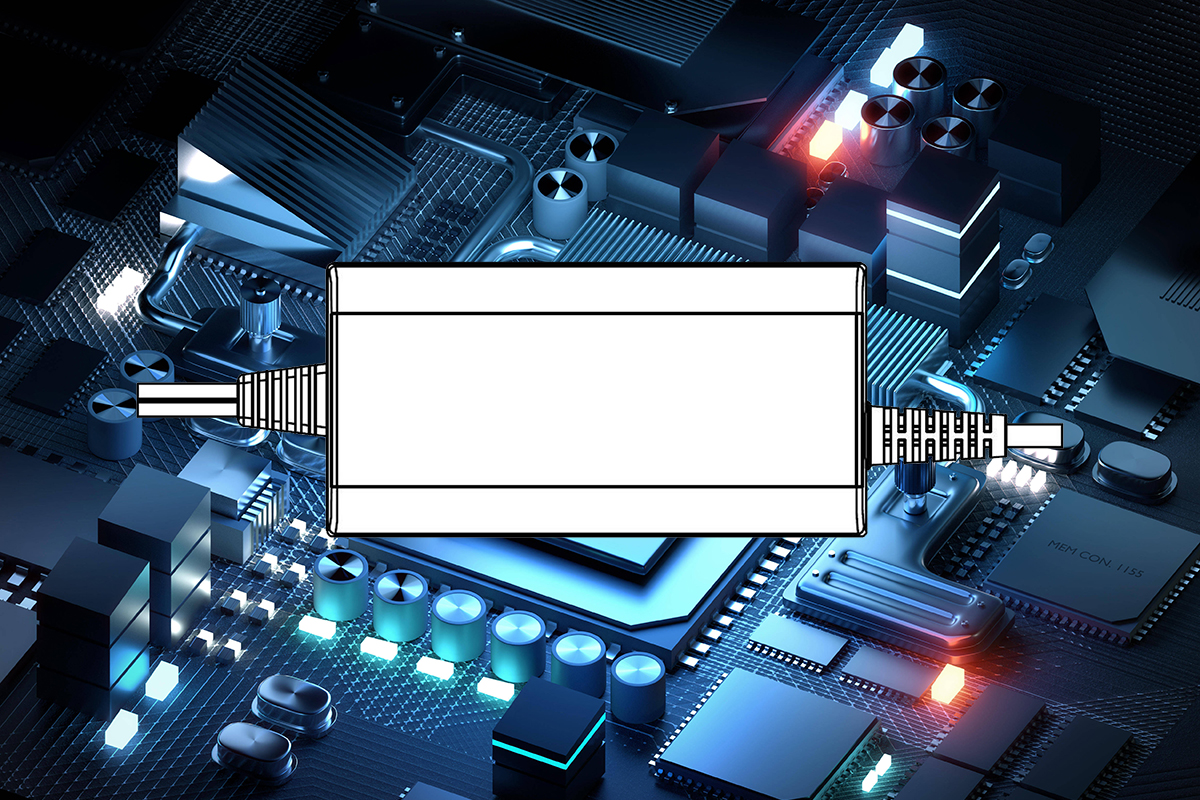
An LED Light Is Only As Good As Its Driver
The development and deployment of light emitting diode (LED) technology across the entire range of lighting applications have been breathtaking over the past few years. Despite the inherent high electro-optical conversion efficiency of LEDs, an LED light is only as good as its driver. The potential of this revolutionary lighting technology can only be unlocked when the performance metrics of LED drivers are consistently matched to the electrical characteristics of the LED light source. An LED lighting system is a synergistic combination of the light source, LED drivers, thermal management systems, and optics. Being the only component that characteristically influences the photometric performance and light quality of the LEDs in a lighting system, drivers play a critical role in more extensive and intensive applications of LED technology.What Is an LED Driver?
An LED Driver is an electronic device which regulates the power to an LED or a string (or strings) of LEDs. LEDs are solid state semiconductor devices impregnated, or doped, with layers to create a p-n junction. When the current flows across the doped layers, holes from the p-region and electrons from the n-region are injected into the p-n junction. They recombine to generate photons which we perceive as visible light. The conversion from current to light output is nearly linear, increasing the input current allows more electrons and holes recombining in the p-n junction and thus more photons are generated.In contrast to conventional light sources that runs directly from an alternating current (AC) power supply, LEDs operate on DC input or modulated square wave input because the diodes have polarity. An input of the AC signal will cause an LED to only light up approximately half of the time when the AC signal is the correct polarity and immediately go out under negative bias. Hence, a constant supply of DC electrical current at a fixed output or a variable output within an allowed range must be applied to an LED array for stable, non-flickering lighting.
LED drivers provide an interface between the power supply (line) and the LED (load), converting the incoming 50 Hz or 60 Hz AC line power at voltages such as 120 Volts, 220 Volts, 240 Volts, 277 Volts or 480 Volts to the regulated DC output current. There are drivers designed to accept other types of power sources as well, e.g., DC power from DC micro-grids or Power over Ethernet (PoE). An LED driver circuit should have immunity against voltage spikes and other noise on the AC line within a predetermined design range while also filtering out harmonics in the output current to prevent them from affecting the output quality of the LED light source. The driver is not merely a power converter. Some types of LED drivers have additional electronics to enable precise control of the light output or to support smart lighting.
Constant Current or Constant Voltage?
An electrical circuit that regulates the incoming power to provide a constant-voltage output has typically been referred to as a power supply, whereas an LED driver in the strict sense refers to an electrical circuit that provides a constant current output. Today, "LED driver" and "LED power supply" are very ambiguous terms that are being used interchangeably. Despite the terminological ambiguity, we can't afford to neglect the intrinsic differences between the constant current (CC) and constant voltage (CV) circuit schemes for LED load regulation.Constant current LED drivers provide a constant current (e.g., 50mA, 100mA, 175mA, 350mA, 525mA, 700mA, or 1A), regardless of the voltage load, to an LED module within a specific voltage range. The driver may power a single module with LEDs connected in series or multiple LED modules connected in parallel. Series connection is preferred in CC circuit architectures because it ensures all the LEDs have the same current flowing across their semiconductor junctions and the light output is uniform across the LEDs. Driving multiple LED modules in parallel requires a resistor in each LED module, which leads to lower efficiency and poor current matching. Most CC drivers can be programmed to operate over an output current range for precise pairing between the driver and a specific LED module. Constant current LED drivers are used when light output should be independent of the input voltage fluctuation. They are found in many types of general lighting products, such as downlights, troffers, table/floor lamps, street lights and high bay lights, for which high current quality and precise output control are the priority. CC drivers support both pulse-width modulation (PWM) and constant-current reduction (CCR) dimming. Operating a power supply in a CC mode usually requires overvoltage protection just in case an excessive load resistance is encountered or when the load is disconnected.
Constant voltage LED drivers are designed to operate LED modules at a fixed voltage, typically 12V or 24V. Each LED module has its own linear or switching current regulator to limit the current in order to maintain a constant output. It is generally preferred to provide a constant voltage supply to multiple LED modules or fixtures connected in parallel. The maximum number of LEDs or LED modules and the forward voltages across them must not exceed the DC electrical energy power supply. The CV circuit must tolerate the power dissipation when the load goes short circuit. The current limiters typically have thermal shutdown to protect the circuit when a voltage higher than the maximum allowable voltage is placed across the current limiter. CV drivers are often used in low voltage LED lighting applications that demand ease of group connection in parallel control, e.g., driving LED strip lights, LED sign modules for lightboxes. Constant voltage drivers can only be PWM dimmed.
Switched-mode Power Supply (SMPS)
As LEDs are very sensitive to current and voltage fluctuations, one of the most important roles of an LED driver is to reduce variations in forward voltage across the semiconductor junction of the LEDs. Switched-mode power supplies operate by modulating an electrical signal using one or more switching elements such as power MOSFETs at a high frequency thereby generating the predetermined magnitude of DC power under supply voltage or load variations. Switch-mode converters used in LED drivers require energy to be stored as current using inductors and/or as voltage using capacitors so as to maintain the output current or voltage on the load during the on/off switching cycle. An AC-DC SMPS LED driver rectifies AC power into DC power which is then converted into DC power capable of driving the LEDs properly.For the switched-mode power conversion in LED drivers, various circuit topologies are available to support the LED load requirements. Among all SMPS topologies, buck, boost, buck-boost, and flyback are the most commonly used types.
Also known as a step-down converter, a buck circuit regulates input DC voltage down to a desired DC voltage using a number of current control methods, including synchronous switching, hysteretic control, peak current control, and average current control. The buck topology is designed for mains-powered LED drivers which are required to drive a long string of LEDs, with the load voltage kept under the supply voltage. Buck circuits are also frequently found in low voltage applications where the input supply voltage is relatively low (e.g., 12 VDC for automotive lighting) and just one LED is being driven. The buck topology allows for circuit design with fewer component counts while maintaining a high efficiency (90–95%). However, the load voltage of a buck circuit must be less than 85% of the supply voltage. Moreover, buck LED drivers do not offer isolation between the input and output circuits.
A boost converter is designed to step up the input voltage to a higher output voltage by about 20% or more. Boost circuits generally require one inductor and operates in either the continuous conduction mode (CCM) or discontinuous conduction mode (DCM), as determined by the waveform of the inductor current. Low-power boost converters can use a charge pump, rather than an inductor, which uses capacitors and switches to raise the output voltage above the supply voltage. Inductor-based converters offer the advantage of low component counts and high operational efficiencies (greater than 90%). The disadvantage of this topology is that it offers no isolation between the input and output circuits. Boost converter outputs a pulsed waveform and thus requires a large output capacitor to reduce the current ripple. PWM dimming is challenging with the large output capacitor as well as the closed-loop control which demands a large bandwidth to stabilize the converter.
Buck-boost converters can provide an output higher or lower than the input voltage, making them ideal for applications where the input voltage rises and falls with a large variation (no more than 20%). Input voltage fluctuations of this type usually occur in battery-powered lighting applications, e.g., vehicle-mounted lighting for construction and agricultural machinery (forklifts, tractors, harvesters, diggers, snow ploughs, etc.) as well as trucks and buses. Two types of converters often found in buck-boost applications are known as SEPIC (single-ended primary inductance converter) and Cuk. The SEPIC converter is characterized by the use of two inductors, preferably a dual-winding inductor which has a small footprint, low leakage inductance, and the ability to increases the coupling of the windings for improved circuit efficiency. In a SEPIC architecture, the boost section provides power factor correction (PFC) and the buck section produces a voltage to be the same as, lower, or higher than the input voltage, while output polarity of both sections remains the same. The Cuk topology combines the continuous output current of a buck and the continuous input current of a boost, which gives the Cuk the best EMI performance and allows the capacitance to be reduced as needed. The buck-boost converter is a non-isolated driver circuit. Like boost converters, buck-boost converters require overvoltage protection to prevent damages from excessively high voltage in case of an open-load condition.
A flyback switching circuit is a discontinuous conduction mode converter which provides AC mains isolation, energy storage, and voltage scaling. It is very much like a buck-boost converter, but with the inductor split to form a transformer. The flyback transformer with at least two windings not only provides complete isolation between its input and output circuits, but also allows for more than one output voltage in different polarities. The primary winding is connected to the input power supply, the secondary winding is connected to the load. Magnetic energy is stored in the transformer while switch is on and at the same time the diode is reverse-biased (i.e., blocked). When the switch is off, the diode is forward-biased and magnetic energy is released by current flowing out of the secondary winding. Some flyback circuits use a third winding, called a bootstrap or auxiliary winding, to power the control IC. More accurate control of the average voltage across the capacitor, which is used to maintain current flow in the LED load when the converter is on the first step, requires isolated feedback, usually via an optocoupler. Flyback swiching circuits can be designed for a very wide range of supply and output voltages, with isolation from dangerously high voltages. However, these circuits are less efficient (75 - 85%, higher efficiency is possible by using expensive parts).
Linear Power Supply
A linear power supply uses a control element (such as a resistive load) which operates in its linear region to regulate the output. In this type of LED driving circuits, the voltage flowing through a current-sensing resistor is compared to the voltage reference in a feedback loop to produce the control signal. A controller which is operated in a linear region of the closed loop feedback system adjusts the output voltage until the current flowing through the sensing resistor matches the feedback voltage. The current delivered to an LED string is thus maintained as long as the forward voltage does not exceed the dropout-limited output voltage. Linear drivers provides only step-down conversion, which means the load voltage must be kept lower than the supply voltage. If the load voltage is higher than the supply voltage or the supply voltage has a wide variation, a switching regulator is needed.AC mains-powered applications, which has demanding requirement for voltage regulation, typically employ switched linear regulators to drive LED lamps with a long string of LEDs wired in series. Switched linear regulators are combinations of multiple linear regulators which are either integrated or cascaded in a modular form. Typically designed in surface-mount IC packages, these linear regulators are used to intelligently adjust the number of load connected LEDs in a string during a power line cycle so that the load voltage matches the instantaneous AC mains voltage.
Linear LED drivers provide an extremely simplified solution which eliminates the need for bulky and costly coils, capacitors, and the reactive (e.g., inductive and/or capacitive) input EMI/EMC filter elements. A significantly low parts count and the use of solid state components allow the switched linear regulator to be downsized to a compact IC chip. This makes linear drivers a competitive candidate for LED lamps of which cost and physical size are important design considerations. With the ability to generate a substantially resistive dimmer load which is similar to an incandescent lamp, linear LED drivers have generic compatibility with legacy phase-cut (TRIAC) dimmers that were designed for dimming resistive loads.
Characterized by its cost competitiveness, EMI/EMC immunity, small footprint and design simplicity, the linear driving topology is gaining a rising interest in the industry. However, linear drivers are struggling with their inherent disadvantages that hold them back from entering mainstream applications in quite a few product categories.
- A linear LED driver can be of low efficiency, when the supply voltage runs substantially higher than the load voltage.
- The excess power is released as heat energy, resulting increased thermal stress on the driver circuit and very likely on the LEDs as well if heat is not efficiently dissipated.
- The limitation of having to keep the load voltage lower than the supply voltage within a certain range leads to a further disadvantage of only allowing a restricted supply voltage range.
- Linear drivers available on the market are dominantly low cost circuits which give no special consideration on flicker elimination.
- The non-isolated topology provides no electrical isolation from the AC mains supply.
Switched Vs. Linear
The design of an LED driver involves many compromises. The selection between SMPS and linear drivers has to take cost, efficiency, control, lifespan, dimming, size, power factor, flicker, input/output, AC mains isolation, and various other factors into consideration.Switching power supplies are obviously more efficient than the linear ones because of their "0/1" (ON/OFF switching) modulation. They can be designed to deliver high power efficiency as well as flicker-free illumination while maintaining a high power factor and low total harmonic distortion (THD). While linear LED drivers have been envisioned to be a prospective LED driving solution, SMPS is, for the foreseeable future, still the preferred LED driving solution for applications where efficiency, lighting control, light quality, and electrical safety are of paramount concern. In particular, the digital controllability of SMPS drivers, which are equipped with smart sensor technology and wireless connectivity, promises to enable a variety of Internet of Things (IoT) applications. Digital modulation allows encoding the data in binary for high-speed optical wireless communication (LiFi), which vastly expands the application potential of SMPS drivers.
Nonetheless, the captivating features of SMPS drivers are achieved at the expense of their dependence on bulky, expensive and unreliable reactive components, such as transformers, inductors, and capacitors. High-speed switching operation causes much noise, thus leading to a relative high level of electromagnetic interference which has to be filtered and screened using additional circuits. These additional circuits can tremendously increase the physical sizes and double the overall cost of the LED driver.
The largest disadvantage of SMPS drivers, which is also the most attractive feature of linear drivers, is their reliability. An SMPS driving circuit uses a large number of components including filters, rectifiers, power factor corrector (PFC) circuits, etc. The complex design may degrade circuit reliability. Widespread use of aluminum electrolytic capacitors in the PFC as an energy-storage component introduces the biggest concern about the reliability of an SMPS driver. Electrolytic capacitors are known for their high-capacitance value and high-voltage rating. Nevertheless, the electrolyte in the capacitor will evaporate over time. The evaporation rate linearly correlates to temperature. High temperature will accelerate electrolyte evaporation, which causes a decrease in capacitance and an increase in ESR (equivalent series resistance). Increased ESR translates to high output voltage ripple and noise. And the capacitor eventually fails when electrolyte dries out, leading to the premature failure of the entire lighting system. High-speed switching operation can produce electromagnetic interference (EMI) which adversely affects the surrounding circuit elements. This poses an additional design challenge to overcome. The uses of a noise filter leads to an increase in volume and weight as well as manufacturing cost.
On the other side, linear drivers do hold a great potential owing to the previously mentioned advantages. They typically outlive SMPS drivers, simplifies lamp design, and delivers cost, and reduce the BOM significantly. However it's challenging to design a linear driver with conversion efficiency and flicker mitigation comparable to SMPS circuits. This technology is currently being abusively used. The majority of the lighting manufacturers take it as a low cost driving solution only. While it's acceptable to employ linear drivers in LED luminaires for applications where high quality light and AC mains isolation aren't a top priority (e.g. outdoor lighting), some manufacturers are attempting to incorporate this low cost LED driving solution in the visually demanding, safety sensitive indoor lighting applications without improving the driver’s output quality (flicker control) and enhancing the electrical safety and heat dissipation of the lighting system.
DOB
Driver-on-board (DOB) is a typical implementation of the linear driving topology. Also called an AC LED light engine, the DoB LED module accommodates the LEDs and all the driver electronics on a metal-core printed circuited board (MCPCB). DOB technology takes advantage of the MCPCB-mountability of the high voltage driver ICs (switched linear regulators). Unlike the SMPS driver circuitry which has to be mounted on a routed FR4 PCB, these surface-mount driver ICs can be soldered to the LED-mounted MCPCB without circuit routing. This eliminates entirely the need for a dedicated driver assembly and thus allows for a compact form factor. Another benefit of DOB design is that the excellent thermal conductivity of the MCPCB can facilitate rapid dissipation of heat generated due to the inefficient conversion of a linear driver.Power Utilization
The power processing that goes on inside an SMPS usually causes its power drain to be uneven due to current pulse modulation. The way that switching regulators draw pulses of current from the utility power grid can produce kinks and distortions in the power line current waveform as well as trip fuses and circuit breakers at power levels lower than power line capability. The presence of these harmonic distortion and nonlinear loads can lead to various problems such as overheating of neutral conductors and distribution transformers, failure or malfunction of the power generation and distribution equipment, and interference with communication circuits, etc. From a utility point of view, these damaging disturbances from downdream electrical equipment must be prohibited. Therefore utility companies have regulatory requirements on the power factor (PF) and total harmonic distortion (THD) of electrical equipment, including line-powered LED luminaires.Power factor is the ratio of power utilized to power delivered and is expressed as a number between 0 and 1. A purely resistive loads has a power factor of 1 because draws current exactly in phase with the line voltage. Nevertheless, the reactive elements such as capacitors and inductors of an LED driver draw an additional reactive current which is difficult to measure and therefore impossible for the utility companies to collect revenue from. Most importantly, this reactive power will cause the delivered power (apparent power) larger than the power actually required by the LED luminaire. This can cause the utility's infrastructure to operate above capacity and can incur damage potential if no measure is taken to protect the infrastructure from being overloaded by the additional reactive power. The closer the PF is to 1, the more closely matched the current and voltage waveforms are. As the PF decreases, more power is wasted in the form of reactive power. In the commercial and industrial sectors, utilities will often surcharge end-users who operate with low-PF electrical equipment to compensate increased generation and transmission cost.
The power factor of an LED lamp or luminaire has become a specification requirement in many markets. The EU Directive requires an LED product with a power consumption of more than 25 W to have a PF higher than 0.9. In the U.S., both Design Light Consortium (DLC) and Energy Star have PF regulations similar to Europe. The State of California has clear regulation for PF value which has to be greater than 0.9 for all power levels of residential and commercial LED lighting. In order to meet regulatory PF values, line-powered LED drivers designed for AC mains applications must employ some form of power factor correction to maintain a high power factor over a wide input voltage range. A power factor correction (PFC) circuit is typically used to minimize the reactive power and maximize the available power from the source and distribution cabling. PFC circuits, which include active and passive PFCs, shape and time-align the input current into a sinusoidal waveform that is in phase with the line voltage.
Total harmonic distortion (THD) is often brought up in the same breath with the issue of a low PF. THD is a measurement of distortion in the current waveform caused by non-linear electrical loads such as rectifier loads. Distorted current waveforms can reduce the PF and create harmonic distortion as well. Harmonic distortion also occurs when the load draws a current that does not resemble a true sinusoid. THD is represented as a percentage. The lower the value, the better. High THD can cause issues within the power distribution equipment. So it's important that LED drivers meet regulatory THD values (typically less than 20%) over the entire input voltage range. THD is suppressed by the power factor correction circuitry which must effectively shape the input current to ensure minimal energy at higher frequencies is generated.
Both PF and THD can be affected by dimming. Therefore, it is necessary to have PF and THD measured at full and dimmed outputs.
Dimming Control
The transition from traditional lighting technology to solid state lighting is driven by the need for greater efficiency, control and interaction. At the heart of lighting control is dimming technology which is an integral functionality of light management systems. One of the advantages to LEDs is the ability to respond instantaneously to changes in power input which is regulated by the LED driver. The dimming performance of an LED driver is increasingly important as lighting becomes more connected and adaptive to user needs and preferences. The most commonly used dimmer-to-driver controls include triac (triode for alternating current), 0-10V, and DALI (Digital Addressable Lighting Interface). Pulse width modulation (PWM) and constant current reduction (CCR) are the most common methods used to dim LED loads from the driver.Phase control dimmers operates by chopping-out portions of the AC voltage cycle to control the light output. Phase control circuits include 2-wire forward phase (leading edge) control, 2-wire reverse phase (trailing edge) control and 3-wire forward phase (leading edge) control. Phase control dimming is often used in retrofit applications where pulling new or additional branch circuit wiring or back-end control wiring can be complicated and expensive. However, the LED driver must be designed to recognize and respond to the voltage signals from the dimming circuit. Failing to interpret a variable phase angle output from the phase control dimming is likely to produce flicker and reduce the dimming range.
0-10V is a 4-wire (Hot and Neutral, plus 2 low-voltage control wires) dimming method and is sometimes referred to as 1-10V dimming as most typical 0-10V dimmable drivers can only be dimmed from 100% (10V) down to 10% (1V), and 0V turns the lamp off. In this method, the driver is the current source for the DC signal and is thus is reliable with dimming occurring in the driver. The control circuit sends low voltage control signals to adjust an input to the driver by varying the voltage between 1V and 10V DC. Since the control signal is a small analog voltage, long wire runs can cause a voltage drop and produce a drop in the signal level. 0-10V is a universal control protocol in the lighting industry and finds its popularity in commercial lighting applications. However, the 0-10V dimming standards for architectural applications in the US do not define the value of the minimum light output and address the shape of the dimming curve. This is likely to cause incompatibility between controls and devices from different manufacturers.
DALI, with the ability to provide addressing of individual fixtures and status feedback from the loads, provides great flexibility in lighting control through a 4-wire (Hot and Neutral, plus 2 low-voltage data link topology-free wires) system. DALI is typically used where the control strategy requires the light fixture to respond to more than one controller (e.g., a manual control switch and an occupancy sensor). DALI is a bidirectional protocol and a DALI lighting system can operate up to 64 control points (drivers, dimmers, relays) without using a central control unit. The DALI protocol uses logarithmic dimming which provides 256 steps of brightness with a standardized dimming curve in the range of 0.1% to 100%.
PWM controls the brightness of an LED by varying the duty cycle of a constant current at a pulse rate high enough to be imperceptible to the human eye. The ratio of on time to off time determines the perceived light intensity. Pulse width modulation keeps the forward current constant, which eliminates the concern of color shift and is thus advantageous for applications that requires a consistent CCT over a wide dimming range. PWM dimming is commonly used for both static and dynamic intensity adjustment with white light sources as well as RGB LEDs. In RGB color mixing applications, PWM dimming allows the brightness of the individual sources to be precisely adjusted to deliver the desired color. However, high speed switching can generate electromagnetic interference. PWM drivers cannot be mounted remotely from the light source because the increased transmission distance from the driver to the light source may interfere with the high frequency, time sensitive duty cycles.
CCR or analog dimming adjusts light intensity by changing the DC drive current flowing through an LED. Because the current is changed linearly, CCR is essentially flicker-free. Constant current dimming can also operate over a wider range of light output than typical phase-cut dimming. The disadvantages of CCR include poor performance at low currents (below 10%), color shift of LEDs when dimming the LEDs to 20% of the rated output, and asynchronous response at higher currents due to the droop effect. CCR dimming circuitry can be controlled through a variety of protocols, such as 0-10V, DALI, and ZigBee. CCR and PWM can be combined to provide hybrid dimming so the advantages of both techniques can be harvested.
Flicker Mitigation
Flicker is amplitude modulation of the light output that can be induced by voltage fluctuations in AC mains, residual ripples in the output current provided to the LED load, or incompatible interaction between the dimming circuits and LED power supplies. Flicker can cause other temporal light artifacts (TLAs) which include stroboscopic effect (the misperception of motion) and phantom array (pattern appears when eyes move). TLAs come in both visible and invisible forms. Flicker that occurs at frequencies of 80 Hz and lower is directly visible to the eye, and invisible flicker is the temporal variations occurring at frequencies of 100 Hz or higher. The stroboscopic effect and phantom array will typically occur within a frequency range of between 80 Hz and 2 kHz, their visibility varies in populations. While invisible TLAs is not perceptible to the human eye they can still have a number of negative consequences.Flicker and other TLAs are undesired temporal patterns of light output that can cause eye strain, blurred vision, visual discomfort, reduced visual performance and, in some cases, even migraines and photosensitive epileptic seizures. Therefore they're one of the key considerations in light quality assessment. The intended use of artificial lighting plays a role. Different lighting scenarios may tolerate different level of temporal light artifacts. TLAs may be less of a concern for roadway, parking lot, and outdoor architectural lighting, or other applications where the duration of exposure to artificial light is limited. Artificial light with a high percentage of flicker should not use for both ambient lighting and task lighting in homes, offices, classrooms, hotels, laboratories and industrial spaces. Flicker-free lighting is not only critical for visual tasks that demand precise positioning of the eyes and environments where susceptible populations spend considerable time, it's high desired for HDTV broadcasting, digital photography and slow-motion recording in studios, stadiums and gymnasiums. Video cameras can pick up TLAs the way like the human eye detects these effects.
The key to mitigating flicker lies in the LED driver which is designed to rectify commercial AC power into DC power and filter out any undesirable current ripple. Sufficiently large ripples, which typically occurs at twice the frequency of the AC mains voltage, in the DC current provided to the LED load result in flicker and other visual anomalies at a frequency of 100/120 Hz. Thus the allowed level of ripple current in the LEDs, such as ±15% ripple (a total of 30%), must be defined in LED drivers for various applications where flicker matters. The ripples may be smoothed out by using a filter capacitor. One of the major challenges in driver design is to filter out ripples and harmonics without using bulky, short-lived high voltage electrolytic capacitor on primary side. AC LED engines are inherently susceptible to the flicker phenomenon because the LEDs in fact run from what is essentially the intermediary DC voltage that would be in an SMPS-based LED lighting system. Rapid alteration in polarity gives rise to a flicker in the intensity at a frequency twice the AC sinusoidal frequency. Despite the simplicity in circuit design, additional circuitry is required to effectively reduce the temporal variation in the power supply.
Standards for limiting flicker for different applications are yet to be established. Two metrics were established by IES to quantify flicker. Percent flicker measures the relative change in the light modulation (the depth of modulation). Flicker index is a metric that characterizes the intensity variation over the entire periodic waveform (or duty cycle, for square waveforms). Percent flicker is better known to general consumers. In general, 10 percent flicker or less at 120 Hz or 8 percent flicker or less at 100 Hz is tolerable for most people except for the at-risk populations, 4 percent flicker or less at 120 Hz or 3 percent flicker or less at 100 Hz is considered safe for all populations and highly desired in visually intensive applications. Unfortunately, a large number of LED lamps and luminaires currently supplied on the market have a high flicker percentage. AC LED lights, in particular, come with flicker typically higher than 30 percent at 120 Hz.
Circuit Protection
Depending on the driver topology, circuit design and application environments, LED drivers can run up against load anomalies and abnormal operating conditions such as overcurrent, overvoltage, undervoltage, short circuit, open circuit, improper polarity, loss of neutral, and overheating, etc. Therefore, LED drivers should incorporate protection mechanisms in order to address these challenges.The output voltage of some constant current drivers, especially switching boost converters, can rise too much above the nominal drive voltage due to load disconnection or excessive load resistance. Open circuit protection or output overvoltage protection (OOVP) provides a shutdown mechanism which uses a Zener diode to give feedback and conduct the output current to ground when the output voltage exceeds a certain limit. A more preferred method of open circuit protection is to utilize an active voltage feedback scheme to shut down the supply when the overvoltage trip point is reached.
Input overvoltage protection (IOVP) is designed to relieve the driving circuit from overvoltage stress as a consequence of switching operations/load change on the power grid, lightning strikes nearby, lightning strikes directly on the lighting system, or electrostatic discharge. In AC line applications, slight but sustained overvoltage can cause high currents (energy impulses) in the LED driver and LEDs, which may lead to failure of the LED driver and control interfaces, and the premature aging of the LEDs. A metal oxide varistor (MOV) or transient voltage suppressor (TVS) can be placed across the input to absorb energy by clamping the voltage. A plastic film capacitor, which is typically connected across the AC line to reduce EMI emissions, also helps absorb some of the energy in surge pulses.
LED drivers usually come with a limited level of surge protection from the built-in overvoltage protection circuits. In some applications such as street lighting, additional surge protection devices capable of surviving multiple surges or strikes should be added to the driver to protect downstream components from high surges. The SPD should be rated reduce or discharge high pulse energy of a minimum 10 kV and 10 kA, as per ANSI C136.2.
A short circuit at the load of a linear power supply can lead to overheating but makes no difference to the current supplied to each LED because the current limiting circuits provide automatic short circuit protection. However, in a switching buck regulator, a short circuit will lead to a failure of an LED or the entire module depending on the circuit design. The failure of a single LED usually has minimal impact on the total light output. The change in voltage can be balanced out using a self-adjusting current sharing circuit which still distributes the current equally. On the other side, a short circuit at the load of an LED string can significant affect the total light output. The failure detection mechanism of short circuit protection can be implemented by monitoring the duty cycle. A short circuit typically results in a very short duty cycle.
Overtemperature protection for LED systems include Module Temperature Protection (MTP) and Driver Temperature Limit (DTL). DTC uses an NTC (negative temperature coefficient) resistor to cut back output current when the maximum driver case point temperature in the application exceeds a predefined limit. MTC monitors the temperature of the LED module and is interfaced with the driver which automatically reduce the current to the LEDs when a threshold temperature is detected by the MTC. DTL can also be used as alternative to MTP if the driver TC point and LED module temperature can be correlated.
EMI and EMC
Electromagnetic interference (EMI), also referred to as radio frequency interference (RFI), affects other electrical circuit as a consequence of either electromagnetic conduction or electromagnetic radiation emitted by electronics such as those in LED drivers, CB radios and cell phones. Any LED driver connected to AC mains supply has to meet the radiated emissions standards such as defined in IEC 61000-6-3. In an LED driving circuit, MOSFET switching is usually the main source of EMI. A PCB layout with paths for the switching currents kept short and compact is also important to limit EMI. In some applications an input filter is required to reduce high frequency harmonics and the design of this circuit is critical to maintain a low EMI. The ground plane on the circuit board must remain continuous so as to avoid creating a current loop that causes high levels of EMI to be emitted. A metal screen may be mounted over the switching area to provide an enclosure that stops EMI radiation.Electromagnetic compatibility (EMC) is the ability of a device or system to operate in its electromagnetic environment without yielding EMI that disturbs neighboring equipment or being disturbed by the EMI radiated by neighboring equipment. The EMC performance of the LED driver is often automatically assured by a good EMI design. However, electrostatic discharge (ESD) and surge immunity which are not taken into account in EMI practices also affects the EMC performance.
Safety Considerations
Safety should always remain the number one priority when evaluating a driver and the lighting system it operates. A line-powered LED driver with dielectric isolation, e.g., 1500 V RMS (50 or 60 Hz), from input to output is highly desired. The input/output circuit isolation can only be accomplished with a transformer which has primary and secondary windings with good galvanic isolation. The output voltage must be kept below the 60 VDC safety extra low voltage (SELV) limit as per IEC 61140. However, there's an increasing number of LED lighting products which implement a non-isolated topology for the purpose of cutting cost. The risk of electric shock is a serious concern in LED products driven by low cost linear regulators. These circuits offer no isolation between the input and output circuits, and the electrical insulation of the lighting systems may have not been adequately tested.The issues of creepage and clearance distances must be considered for AC powered products. The creepage distance between primary and secondary circuits must meet the spacing requirements otherwise electrocution or fire can occur. Clearance, which is defined as the shortest distance between two conductive parts, must be factored in to prevent arcing between electrodes caused by the ionization of air. As the sizes of electronic circuits continue to shrink, a good PCB design is essential for a driver circuit to not only reduce EMI emissions, but also reduces creepage and clearance problems.
All electrically-conductive and touchable parts of a line-powered Protection Class I LED driver must be connected to earth. LED drivers designed to operate LED lighting systems for residential and commercial applications are typically listed as Class II. There's no enclosure grounding for class II LED drivers, but all the conductor inside a class II drivers must be dual or reinforced insulated to ensure good insulation between the mains power circuit and the output side or the metal casing of the driver.