Table of Contents Hide
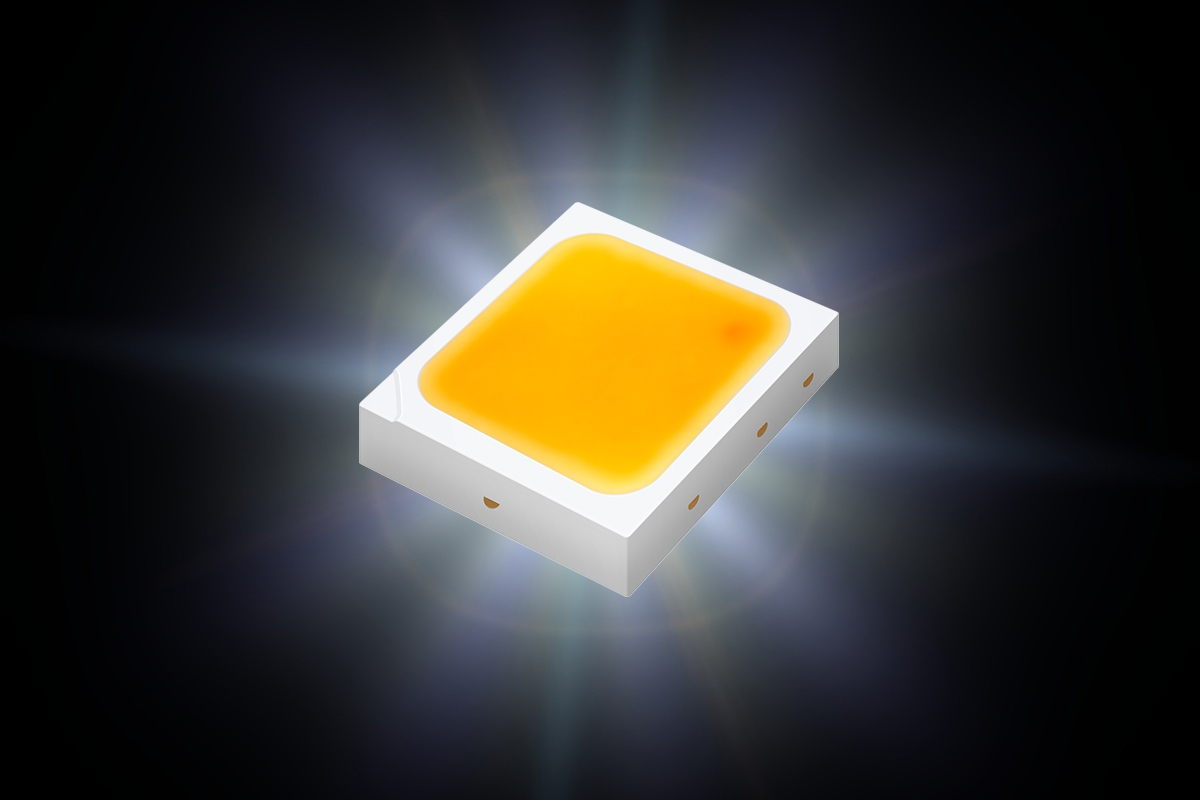
What Are Mid-power LEDs
Mid-power LEDs are LED packages that operate with a power consumption of less than 1 watt but higher than 0.1 watt. Striking a fair balance between cost and efficacy, mid-power LEDs are leading the transition from inefficient, short-lived lighting systems to the cutting edge solid-state lighting systems. An overwhelming majority of interior lighting products are assembled with these discrete packages to deliver uniform illumination as well as design innovation. Featuring a wide beam angle and a compact footprint, mid-power LEDs perform very well in all non-directional solutions ranging from retrofit A-type bulbs, tubular lamps and recessed troffers to flush mount ceiling lights, edge-lit panel lights, linear light fixtures, and light modules designed for architectural lighting systems. Even in the outdoor and industrial lighting sectors some manufacturers are moving towards using cost-effective mid-power LED arrays for applications where beam control is not challenging.Architecture of Phosphor-converted (PC) LEDs
Mid-power LEDs are generally surface-mount PLCC type packages. PLCC is short for plastic leaded chip carrier. Such a surface-mount device (SMD) consists of one or more semiconductor chips (or dies) which are either glued or soldered onto a lead frame. The lead frame, which can be made from a copper alloy or a nickel iron alloy, is designed to provide electrical connection, thermal dissipation and light reflection for the LED die. It is plated with silver, gold, or other metals to provide high light reflectivity and enhance electrical conductivity. The positive and negative electrodes of the LED die are connected to the anode and cathode leads by wire bonding, respectively. Modern mid-power LEDs are also known as Quad Flat No-lead (QFN) packages because most mid-power LEDs are designed with anode and cathode pads on the base of the package rather than the traditional leads formed along the perimeter. In the last stage of the packaging process, the lead frame is molded in a plastic housing wherein a cavity is formed. The cavity is filled with an epoxy resin or silicone-based polymer which is used as the matrix for phosphor encapsulation. SMD LEDs are available with a variety of form factors. The most popular ones are 2835 (3.5 x 2.8 mm) and 3030 (3.0 x 3.0 mm), with 3014 (3.0 x 1.4 mm) and 5630 (5.6 x 3.0 mm) and other sizes being used in various niche applications.Die Fabrication
In phosphor-converted (PC) LEDs, the epitaxial layers of the LED die are typically made from gallium based crystals such as indium gallium nitride (InGaN). InGaN has gained favor over other semiconductor materials because its direct bandgap allows efficient optoelectronic applications. Today, the highest efficiency white LEDs are made from InGaN. InGaN LEDs can be made to deliver internal quantum efficiencies of greater than 70%, external quantum efficiencies of greater than 60%, and luminous efficacies larger than 200 lm∕W.InGaN epitaxial growth can be made on sapphire, silicon, silicon carbide, or gallium nitride. Today's LEDs are almost exclusively fabricated on the sapphire substrate since sapphire is the most cost effective material to support a relatively high quality GaN epitaxial growth. However, heteroepitaxial growth of GaN on sapphire produces greater than 13% lattice mismatch, which results in a high dislocation density in the epitaxial layers. A high dislocation density means more dark spots and less luminous efficacy. One the other side, silicon carbide (SiC) has 4.5x better lattice match to GaN than sapphire and thus enables more light extraction. The disadvantages associated with SiC is that the physical characteristics of SiC pose significant processing challenges.
A more sophisticated approach is to grow GaN on GaN. GaN-on-GaN technology fundamentally addresses epitaxial constraints such as lattice mismatch and CTE mismatch. As a result, a high radiative efficiency can be achieved and very thick layers of GaN can be epitaxially grown for the fabrication of high breakdown voltage devices.
LED Packaging
LED packaging is designed to provide mechanical support, electrical connection, thermal management, spectral transformation, and optical control to the LED dies. As such, packaging represents the single-largest opportunity for performance and reliability improvement. With that said, it is not surprising that most LED failures are associated with LED packaging in a way or another. Depending on application and design requirements, the selection of the packaging material is of crucial importance.Lead frame plating
Light reflection and chemical stability are the foremost characteristics to be considered when choosing a plating material for lead frames. Silver has high reflectance across a large portion of the visible spectrum and is therefore preferred when light output is a priority. However, silver has a high reactivity with sulfur-containing compound. Exposure to corrosive gases such as hydrogen sulfide may lead to formation of silver sulfide which is not electrically conductive. In this case an intermittent or open wire bond stitch and reflectivity degradation of the lead frame will likely occur. In contrast, gold plating provides the lead frame with higher chemical stability, especially against sulfur. However, the light reflectivity of gold-plated lead frames is lower as compared with silver-plated lead frames.Wire bonding
The electrical connection between the LED die and lead frame is made through a solid phase welding process known as wire bonding. Among two major variations of the wire bonding process, ball bonding has higher productivity and more advanced capabilities than wedge bonding. Bonding wires can be made of gold, copper, silver, or aluminum. With its high resistance to surface corrosion and high productivity through the thermosonic bonding process, gold bonding wires are principally used as an interconnection material in semiconductor packaging. However, the creeping rise in the price of gold resulted in a major transition from ball bonding with gold wire to the use of copper and other less expensive bonding metals. Copper rapidly gained a foothold as an interconnection material in LED packaging for its low cost, superior electrical and thermal conductivity as well as greater mechanical stability than gold wires. Despite that copper offers a higher reliability at elevated temperatures, it's subject to oxidation at relatively lower temperatures. This makes copper inherently more difficult to bond. Pure copper wires are coated with palladium to mitigate corrosion cause by oxidation, but this increases overall cost of production. Silver bonding wires have has emerged as another choice for their good electrical and thermal conductivity. Silver has lower melting points and better corrosion resistance than copper. The challenges in using silver bonding wires is that they need to be alloyed to eliminate the silver migration phenomenon. Alloying comes added electrical resistance and causes ball formation difficulties in room temperatures.Encapsulation
The matrix for phosphor encapsulation is expected to present characteristics of high transparency, refractive index, chemical stability, thermal stability, hermeticity, and resistance to UV radiation. Epoxy resin is characterized by low gas and humidity permeability, good thermal expansion coefficient match to LEDs, excellent miscibility with phosphor powder and good mechanical stress protection. However, epoxy resin has limited photostability and is susceptible to thermal oxidation and degradation. Undesired discoloration happens faster under high temperatures. This disadvantage leads to eventual replacement of epoxy encapsulations by silicones or silicone-epoxy hybrids. Silicone has higher thermal stability, excellent moisture/UV light resistance, high optical transparency, and superior biocompatibility. On the downside, silicone has relatively low tear strength, poor adhesion, high moisture and gas permeability, low glass transition temperature, and a large coefficient of thermal expansion (CTE). A hybrid matrix, which combines certain favorable properties of epoxy and silicone, has thus been developed to provide more durable and optically stable encapsulation.Plastic housing
The plastic housing in which the lead frame is molded into can be made of polyphthalamide (PPA), polycyclohexylenedimethylene terephthalate (PCT), epoxy molding compound (EMC), or silicone based composites. Light reflection, thermal stability and photostability are key considerations in the evaluation process of leadframe housing materials. PPA shows high reflectance (>95%) which but has poor photo and thermal stability. In higher power applications the PPA housing tends to yellow at a fast rate and causes a noticeable color shift in the light emitted by the LED. Compared with PPA, PCT has slightly lower reflectance (93%) and poorer moldability but has higher hermeticity and better resistance to photo oxidation and thermal degradation. Accordingly, PPA housings are generally used in SMD packages with power consumption under 0.4W while PCT housings can tolerate operating at higher power ratings (>0.7W). EMC plastics, which have better thermal performance and photostability than conventional PPA and PCT materials, exhibit high lumen maintenance and color stability at higher temperatures and drive currents than PPA- and PCT-based LED packages. EMC has an initial whiteness (reflectance) of 94.8%. Silicone based composites are a serious competitor to EMC plastics thanks to silicone's superior optical and thermal properties. In addition to high temperature stability and UV resistance, silicone has an impressive 97% initial whiteness and good adhesion to lead frames. Both EMC and silicone housings have to be made using the thermal molding process, which translates to a higher cost than PPA and PCT plastics.LED Efficiency
For PC-LEDs, the transfer of electrical to optical energy involves electroluminescence and photoluminescence, which means the process is always accompanied by losses of both electrons and photons. When electrical efficiency of the driver electronics is not counted, the efficiency of energy conversion from electrical power (W) to optical power (radiant flux in watts) of an LED package is essentially the product of the external quantum efficiency (EQE) and the packaging efficiency. External quantum efficiency is broken down as the internal quantum efficiency (IQE) and photon extraction efficiency (EXE). The packaging efficiency is decomposed into the quantum efficiency of the phosphors, the quantum efficiency in down conversion, and the light extraction efficiency at the package level. The electromagnetic energy within the visible wavelength range of the light spectrum is measured in luminous flux, and luminous efficacy is thus calculated by comparing the total lumen output with the electrical power consumed.Because of their limited thermal tolerance, the mid-power plastic LED packages cannot operate at high drive currents. Nevertheless, InGaN LEDs have the highest external quantum efficiencies when they operate at relatively low current densities. Furthermore, in the PLCC architecture the reflective cavity (plastic housing) allows high efficiency light extraction. Thus when compared with high power LEDs mid-power LEDs have an inherently higher luminous efficacy which ranges between 170-200 lm/W (4000K 80 CRI). Even so, there's still a huge energy loss in these high efficacy LED packages. More than 50% of the electrical power is dissipated as heat.
While the largest energy loss at the chip level needs to be continuously improved, the more practical opportunity for efficiency improvement is at the package level. As blue photons are down converted to green and red photons at the phosphor layer, a significant spillover of light across the wide red and green phosphor emission bands leads to a substantial Stokes loss. The typical full width half maximum (FWHM) red LED band is 75 nanometers and the FWHM green phosphor band is 100 nanometers at the time of writing. With optical power optimally distributed into narrower bands in the green and red spectral peaks, Stokes loss, which is about 15–25% of the blue light absorbed by the phosphor, can be reduced.
In addition to the inevitable Stokes losses, the phosphor quantum inefficiencies and mixing/scattering/absorption loss are still responsible for a significant fraction of the optical power loss. Utilizing narrow-band phosphors, optimizing the phosphor's quantum efficiency, and minimizing refractive index mismatch between the LED and encapsulant offer room to enhance the overall efficiency of a PC-LED.
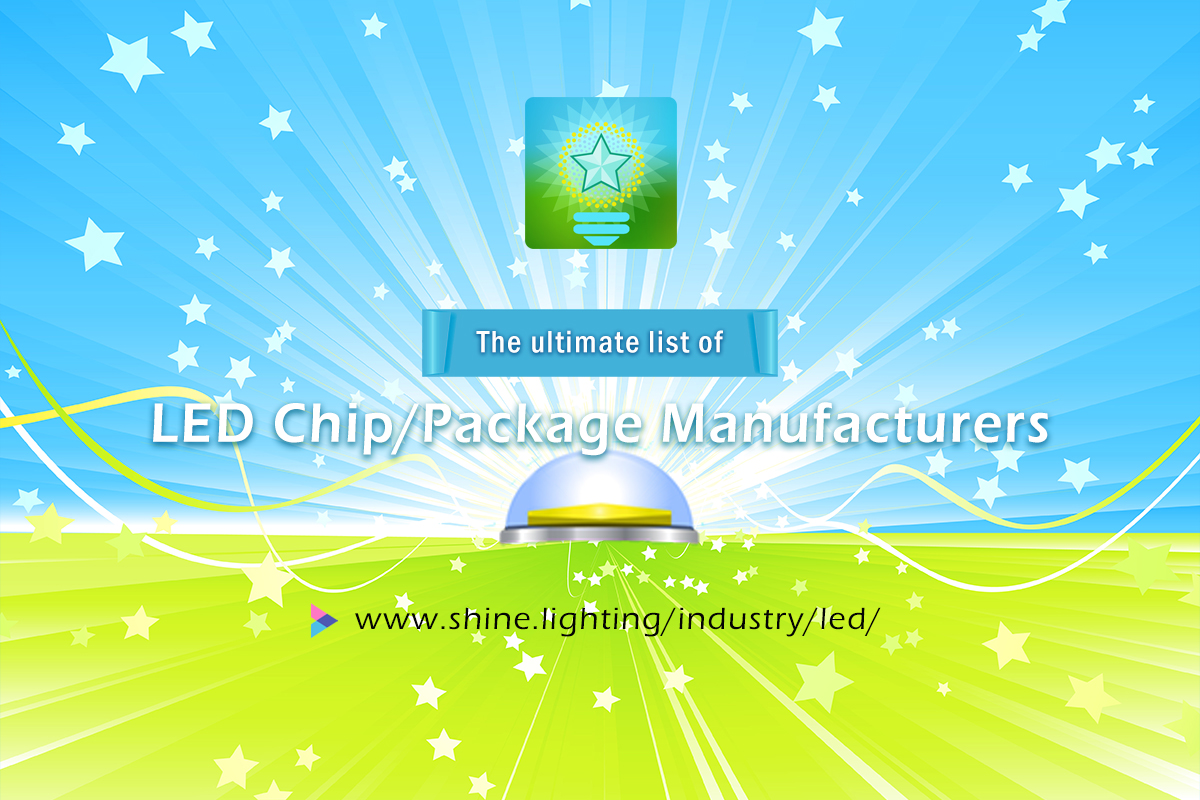
Color Reproduction
The color performance of LED lighting products often gives way to luminous efficiency in today's world which puts too much emphasis on energy efficiency while other equally important specifications of lighting are neglected. The ability of a light source to faithfully reproduce the colors of objects is of critical importance in interior lighting. Color reproduction is expressed using the color rendering index (CRI), a flawed but the only internationally accepted metric for assessing the color rendering performance of light sources. Artificial lighting had once provided superior color rendering performance. Incandescent bulbs have a CRI of 95, which provides a light composition similar to that of the sunlight (100 CRI). The introduction of fluorescent lighting, with a 70-80 CRI, made high CRI lighting a thing of the past. And LED lighting is continuing this tradition, despite the fact that LED technology has the spectral flexibility to produce white light with high color fidelity.The lighting industry seems to have no strong intention to bring the color quality of electric lighting back to what it used to be. This is because currently there is a trade-off between color rendition and luminous efficacy. White light with a high CRI typically requires more emission in the red wavelength range, which means a significant percentage of short wavelength light has to be down-converted to the longer wavelength red light. Since the human eye is more sensitive to shorter wavelengths, reduction in blue wavelength percentage translates to less perceptible light measured in lumens. Meanwhile, phosphor conversion from shorter wavelength photons to longer wavelength photons in a high volume is associated with a huge Stokes energy loss. These two factors explain why the CRI of most LED products is still on the lower end of what is acceptable for residential lighting. The same explanation applies to the abused use of color temperature. Blue-rich, photobiologically risky, circadianly disrupting 6000-6500 K white light is still being introduced for residential lighting in many markets such as China and India. Cool white light has a high percentage of blue and thus more luminous flux is produced. Warm white light, on the other side, has less blue but more red in its light composition.
Mid-power LEDs are the exclusive light source for ambient and task lighting in residential and office settings. Visually demanding tasks, including reading tasks and detail work as well as other color-critical vision tasks require spectrally enhanced lighting. High quality light is also needed to facilitate face-to-face interactions of people and make colors appear natural and realistic in interior environments. Aside from using a down-converter of different phosphor layers, CRI can be improved using a violet-pumped LED source. A recent trend is to use quantum dots (QDs) as down-converters to improve color rendition while minimizing loss in efficiency.
Color Stability
Color stability (also known as chromaticity maintenance, chromaticity shift, or color shift) is a significant challenge for the LED packaging industry. The ability of an LED to maintain its spectral power distribution (SPD) over time depends on package materials, production processes, and construction. Chromaticity shift in LEDs can be triggered and/or accelerated by oxidation of the molding compound in PLCC, loss of phosphor quantum efficiency, phosphor delamination, poor thermal design, high ambient temperature, high optical flux density, or chemical changes and presence of moisture in the phosphors and binder materials. Discoloration of the plastic resin, which can be caused by thermal degradation, photo-oxidation or moisture-polymer reactions, is the dominant chromaticity shift mechanism in mid-power LED packages. Degradation of plastic housing material and lead frame corrosion are another two underlying causes.For many mid-power LEDs, initial chromaticity shift often begins in the blue direction. Blue shift is typically caused by operation above the saturation flux level, oxidation of plastic housing, and leak of blue photons through fractured binder in the phosphor-binder layer. Yellow shift may be resulted from the increase in phosphor quantum efficiency, cracking or delamination of phosphor-binder layer, and surface yellowing of the housing material. Shift in the red direction occurs when there's a decline in the emissions from green phosphors. Reduction in red emissions or phosphor oxidation can lead to green shift.
Lumen Maintenance
Mid-power LEDs are more prone to accelerated lumen depreciation when compared to their high power counterpart. The lumen depreciation modes of LEDs in general coincide with some of the chromaticity shift mechanisms. The plastic architecture of mid-power packages is inherently less resistant to chemical contamination, photo-oxidation and thermal stress than high-power LEDs. Since the high efficacy of mid-power LEDs owes largely to the highly reflective plastic cavity, the degradation and discoloration of this reflective housing can significantly undermine the optical efficiency of the LED packages. The degradation of the plastic material can be accelerated upon interaction with light, moisture, acid, alkaline and/or coating material incompatibility. The metal-plated lead frame can also be a lumen depreciation factor as it can lose reflectivity when corrosive gases or chemicals penetrate through the encapsulation barrier and react with the metal plating.Different types of plastic resin, however, yield different lumen maintenance performance. PPA- and PCT-based LED packages exhibit poor lumen maintenance at higher temperatures and drive currents. These cheap packages have a sizeable share in the entry-level lighting market. EMC and silicone-based LED packages perform remarkably stable at high junction temperature than the low cost PPA- and PCT-based packages. Despite the improvement in thermal stability, a plastic housing (rather than a ceramic substrate) often fails to dissipate very high heat flux densities when the LED is driven hard. Continuous operation at elevated temperature will eventually cause a permanent reduction in light output from the LED. In addition to chip-level thermal stress, down conversion of blue photons creates a large amount of Stokes heat in the phosphor matrix. High thermal stresses will lead to loss of phosphor quantum efficiency accompanied by lumen depreciation and color shift.
Binning
Binning (selection in bins) is an essential part of LED specification. In semiconductor manufacturing processes the process window (such as temperature control in the wafer manufacturing process) is very small and tolerances (e.g. material structure, composition, thickness) are very tight. The difficulty of achieving such a high degree of control renders leads to variations in electrical and optical characteristics within a single production batch or across different production batches of LED packages. Binning is a way of sorting LED packages based on chromaticity (color temperature), lumen output, and forward voltage. The process of separating LEDs based on their characteristics ensures fixture-to-fixture, lamp-to-lamp uniformity and unnoticeable color differentiation by human perception.Chromaticity binning is a serious design consideration for lighting projects where a high level of color consistency is of paramount concern. Currently there are two main methods used to describe the color consistency of LEDs within a bin. The Standard Deviation Color Matching (SDCM) method is based on levels of MacAdam ellipses. A MacAdam ellipse is an elliptical zone within which all colors are indistinguishable by the average human eye. The American National Standards Institute (ANSI) defines color bins as parallelograms and uses the value ∆u'v' to convey the magnitude of the difference. The parallelogram is sized and oriented to approximately enclose a 7-step MacAdam ellipse. The parallelograms are centered on the black body line.
The lighting market has a varied level of color consistency requirements for mid-power LEDs. Most residential lighting products such as LED bulbs and LED tubes have a 7- or 8-step MacAdam ellipse tolerance (7SDCM or 8SDCM), which is obviously on the low end of color control. Commercial, hospitality and high-end residential lighting usually require a more consistent light output of luminaires across an installation. The LEDs incorporated in these luminaires are typically binned to a 3-, 4-, or 5-step MacAdam ellipse.